1. Topological quantum cascade laser
2. High speed quantum cascade laser and quntum well infrared photodetector
3. Monolithic Semiconductor Lasers with Dynamically Tunable Linear-to-Circular Polarization
4. Designer Multimode Localized Random Lasing in Amorphous Lattices at Terahertz Frequencies
5. Tunable quantum cascade laser
1. Topological quantum cascade laser
Topological insulator is a new phase of matter that is conducting on the edges but insulating in the interiors.
It led to the 2016 Nobel Prize in Physics for three of its pioneers. In these years, the concept has migrated from condensed matter physics into
photonics and various structures such as gyromagnetic photonic crystals, helical waveguide arrays, and coupled ring resonators have been demonstrated
to support topologically-protected edge states which is unidirectionally-propagating, backscattering-free and robust. The photonic topological edge
state is intrinsically advantageous for laser emission as it is immune to fabrication defects or disorders. Recently, a new type of lasing utilising
topologically-protected photonic edge states have been reported [1–3]. However, these demonstrations of topological lasers have required an external
laser source for optical pumping, and operated in the conventional optical frequency regime [1–3]. Unlike previous topological lasers that relied on
large-scale features to impart topological protection, in our work, we employ a compact valley photonic crystal and demonstrate the first electrically-pumped
THz QCL based on topologically-protected valley edge states [4]. Lasing with regularly-spaced emission peaks occurs in a sharp-cornered triangular cavity,
even with the introduction of perturbations, due to the existence of topologically-protected valley edge states that circulate around the cavity without
experiencing localisation. The properties of the topological lasing modes are further probed by adding different outcouplers to the cavity. Looking ahead,
it is promising to use the valley degree of freedom in other active photonic devices, and the realization of an electrically-pumped topological
laser points the way towards incorporating topological protection into practical device applications. |
Reference:
[1] M. A. Bandres, S. Wittek, G. Harari, M. Parto, J. Ren, M. Segev, D. N. Christodoulides, M. Khajavikhan,
Science (80-. ). 2018, 359, eaar4005.
[2] G. Harari, M. A. Bandres, Y. Lumer, M. C. Rechtsman, Y. D. Chong, M. Khajavikhan, D. N. Christodoulides,
M. Segev, Science (80-. ). 2018, 359, eaar4003.
[3] B. Bahari, A. Ndao, F. Vallini, A. El Amili, Y. Fainman, B. Kanté, Science (80-. ). 2017, 358, 636.
[4] Y. Zeng, U. Chattopadhyay, B. Zhu, B. Qiang, J. Li, Y. Jin, L. Li, A. G. Davies, E. H. Linfield,
B. Zhang, Y. Chong, Q. J. Wang, Nature 2019, accepted.
|
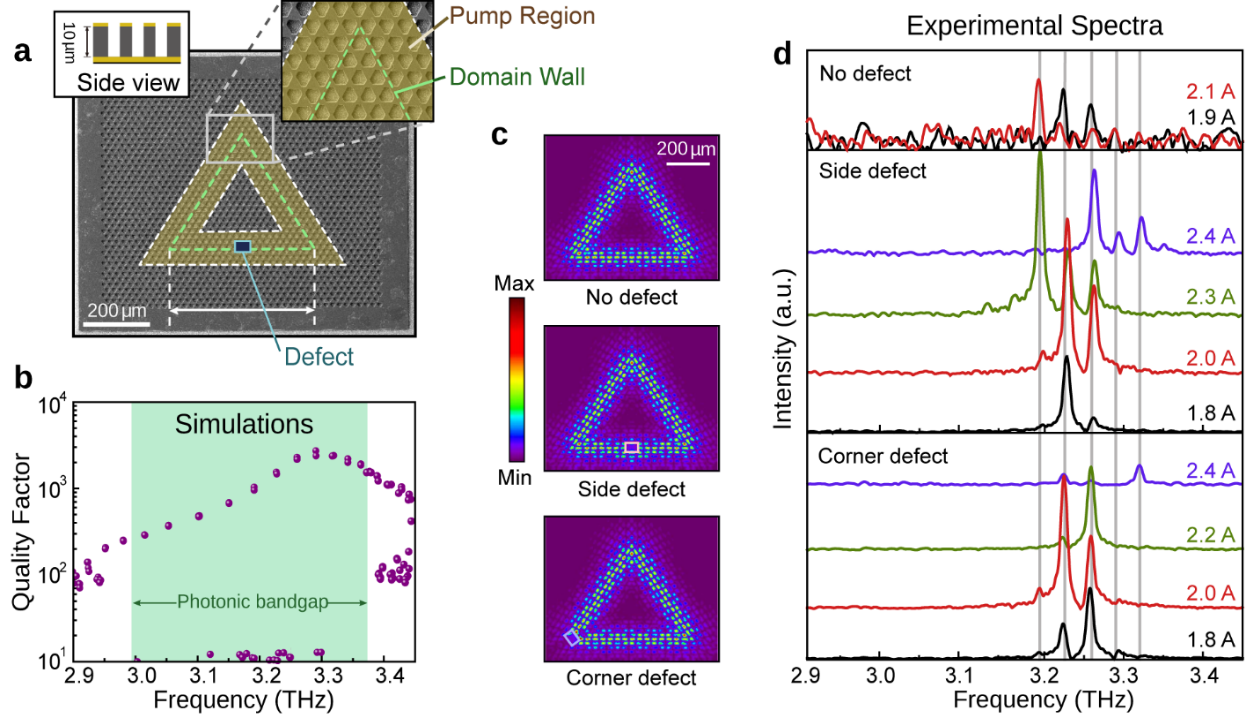
View large figure |
|
|
|
Figure 1. Fabrication and characterization of the topological THz QCL.
(a), SEM image of the THz QCL,
whose optical cavity consists of an in-plane triangular loop of a side length 21a. The shaded yellow area is pumped by electrical injection, while the
other parts are passive. The green dashed line indicates the domain wall. The black rectangle indicates a defect (39 μm×33.5 μm) etched entirely through
the active medium of the THz QCL. Inset: cross-sectional schematic and magnified figure of the domain wall.
(b), Calculated quality (Q) factors of the
structure’s eigenmodes, with realistic material absorption losses (~ 20 cm-1) within the passive region. The shaded area indicates the photonic bandgap
of the valley Hall lattice. (c), Typical eigenmode electric field (|Ez|) profiles at around 3.23 THz, without an outcoupling defect, with a side defect,
and a corner defect. (d), Emission spectra for the QCL without an outcoupling defect (top), with a side defect (middle), and a corner defect (bottom).
Gray vertical lines indicate the peak frequencies of the defect-free QCL, which correspond closely to those of the QCL with a defect. The emission
spectra are vertically offset with increasing pumping intensities for clarity.
|
|
|
|
[1] Zeng, Y. Q., Chattopadhyay, U., Zhu, B. F., et al. “Electrically Pumped Topological Laser with Valley Edge Modes”,
Nature, 578, 246-250, 2020.
[2] Zeng, Y. Q., Qiang, B. & Wang, Q. J. “Photonic Engineering Technology for the Development of Terahertz Quantum
Cascade Lasers”, Advanced Optical Materials 1900573 (2019).
[3] Kim, K., Bittner, S., Zeng, Y. Q., et al. “Electrically pumped semiconductor laser with low spatial coherence
and directional emission", Applied Physics Letters, 115, 071101 (2019).
[4] Cerjan, A., Bittner, S., Constantin, M., Guy, M.,
Zeng, Y. Q., et al. “Multimode lasing in wave-chaotic microcavity lasers”, accepted, Physical Review B (2019).
[5] Li, N., Yuan, H., Xu, et al. “Radiation Enhancement by Graphene Oxide on Microelectromechanical System Emitters for Highly Selective
Gas Sensing”, ACS sensors 4, 2746-2753 (2019).
|
TOP
2. High speed quantum cascade laser and quntum well infrared photodetector
2.1 Tunability of the Free-Spectral Range by microwave injection into a Mid-Infrared
Quantum Cascade Laser
The Free-Spectral Range (FSR) of Quantum Cascade Lasers (QCLs) emitting at 4.7 µm can be tuned through
direct microwave modulation. The intrinsic short carrier lifetime of intersubband transitions, along with an impedance matched electrical
packaging, allow a high-speed modulation up to more than 30 GHz. A significant broadening and flattening of the lasing spectrum are observed
under Radio-Frequency (RF) injection with frequencies close to the round-trip cavity. An accurate analysis of the high resolution spectra of
the laser shows a comb-like regime for both free-running and RF modulated QCLs, if the modulation frequency is within the locking-range of
the device. One of the main advantages of collecting high-resolution mid-infrared spectra, over the plain investigation of the beatnote in
the microwave region, is the access to all the longitudinal modes and thus the accurate measure of the FSR over the whole optical spectrum.
The use of high-resolution spectroscopy provides an in-depth and comprehensive analysis of lasing spectra under microwave modulations. |
|
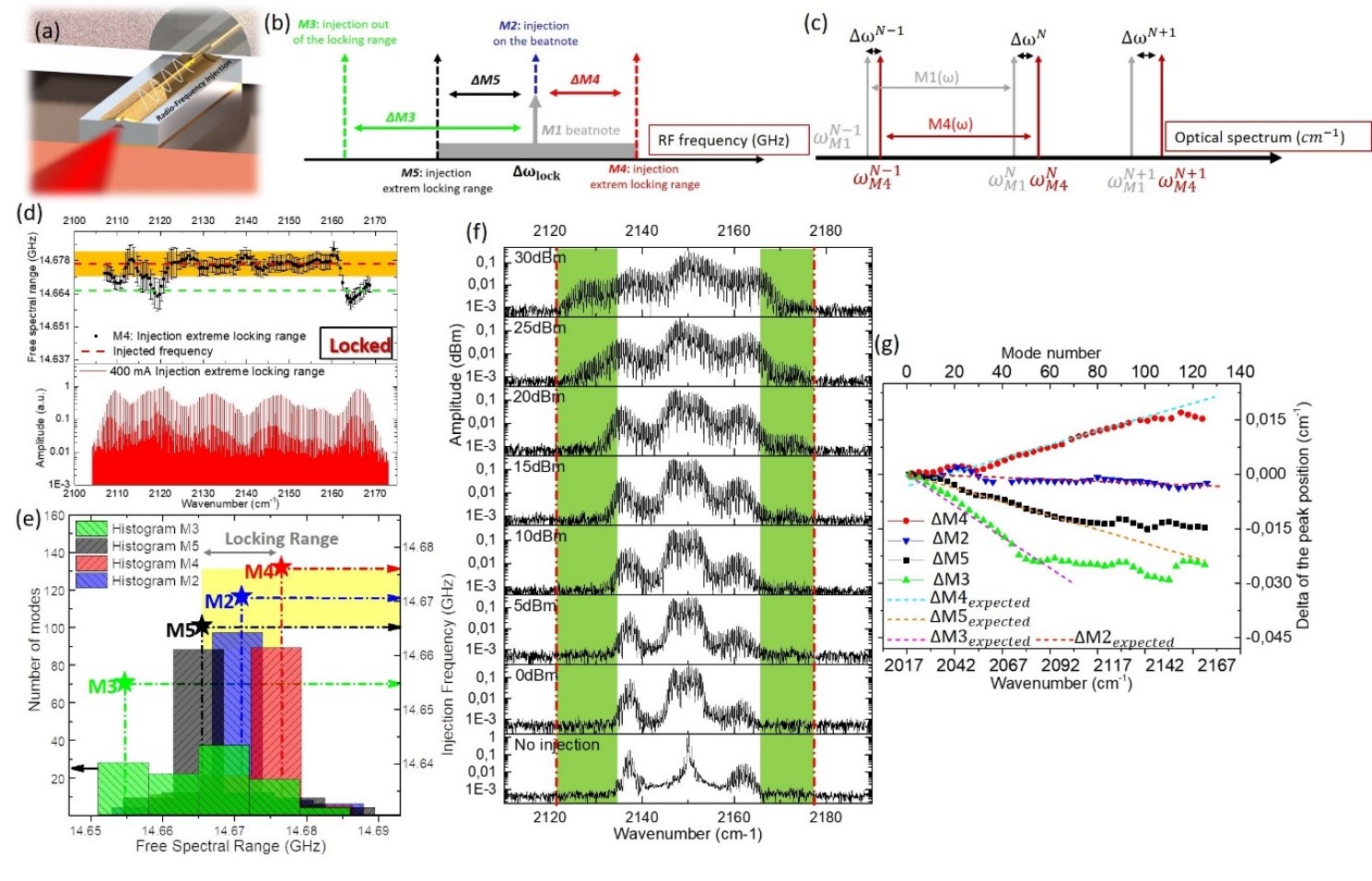
View large figure |
|
|
|
Figure 1.
(a), Artistic representation of the QCL lasing with the RF injection.
(b), Illustration of the measurement M1 without and M2, M3, M4 and M5 with RF injection at different
frequencies in and out of the locking-range represented in grey.
(c), Representation of the variation Δω of the peak position between the free-running spectrum (grey) and injected spectrum (red).
(d), Analysis of the variation of the FSR over the spectra of a QCL emitting at 4.7 µm driven by a current of 400 mA
with RF injection at the extremity of the locking-range.
(e), The right axis represents the injection frequency as a function of the average FSR measured over a
stable area of the spectrum and the left axis is the histograms of the FSR repartition for each measurement. The yellow area represents the locking-range of the device.
(f), Evolution of the spectrum for several power injections for a 4 mm QCL at 4.7 µm with a current of 300 mA corresponding to 1.6 J_th.
(g), The variation of the peak position between the spectra injected (M2, M3, M4 or M5) and the spectrum non-injected taken as reference
(M1) with the expected slope in dashed line (ΔM2, ΔM3, ΔM4, ΔM5).
|
|
|
|
[1] Rodriguez, E., et al. “Tunability of the Free-Spectral Range by microwave injection
into a Mid-Infrared Quantum Cascade Lasers.”,
submitted, Laser & Photonics Reviews (2019).
TOP
|
2.2 Room temperature, wide-band Quantum Well Infrared Photodetector for microwave
optical links at 4.9 µm wavelength
High-speed room temperature quantum well infrared photodetectors (QWIPs) at λ ~ 4.9 µm have been
realised in a strain compensated In0.1Ga0.9As/Al0.4Ga0.6As heterostructure grown on a GaAs substrate. The high-speed properties at room
temperature have been optimised by using a specifically designed air-bridge structure which greatly reduces the time constant of the
effective RC circuit, thus allowing transmission and detection of high-frequency signals. By modulating a high-speed quantum cascade
laser (QCL) centred at λ ~ 4.7 µm we were able to record a modulation of the photocurrent up to ~26 GHz, which is limited by our setup.
At 300 K and under a bias voltage of –5 V our device shows high responsivity and detectivity of 100 mA/W and 1 x 107 Jones,
respectively. The developed high-performance QWIPs at this wavelength are highly promising for optical heterodyne measurement,
high-speed free space communications in microwave optical links and frequency comb QCLs characterisations.
|
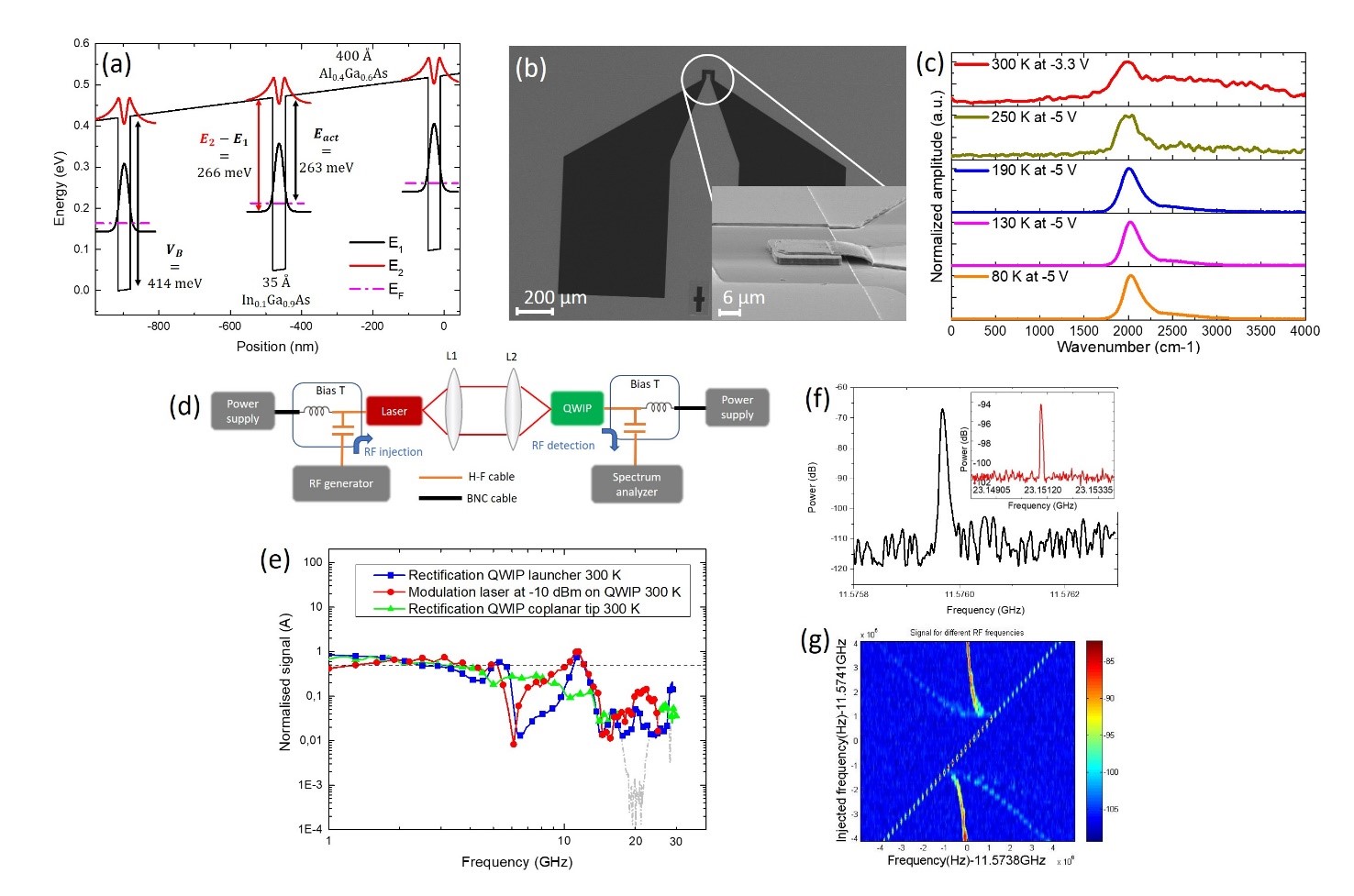
View large figure |
|
|
|
Figure 1.
(a), Band-structure of the developed qauntum well infrared photodetector (QWIP) with 3 quantum wells (QWs) active regions at a bias voltage of 2 V.
The most relavant physical parameters of the sructure are indicated in the figure,.
(b), SEM picture of the top view of the QWIP (The inset: zoom-in of the active region with the air bridge).
(c), Spectra of the QWIP at several different temperatures up to 300 K.
(d), The schematic illustration of the setup used to investigate the high-speed modulation of the QWIP.
A high-speed direct-modulated quantum cascade laser (QCL) is used in the experiment.
(e), Photoresponse of the QWIP with the QCL modulated at –10 dBm at 300 K (red line + symbol) at a bias voltage of –4 V.
The rectification measurements of QWIP using the cryostat setup (blue line + symbol) and using the coplanar tip configuration (green line + symbol)
with in dashed grey line the resonances due to parasitic effects of its electrical circuit. The black dashed line represents the value at -3 dB.
(f), The first harmonic of the beatnote, corresponding to the 3 mm laser, is collected on the QWIP at 300 K. The inset: the second harmonic of the beatnote at 23.15 GHz;
injection-locking observed on the QWIP at 300 K by
(g), tuning the injection frequency on a 3 mm laser.
|
[1] Rodriguez, E., et al. “Room temperature, wide-band Quantum Well Infrared Photodetector
for microwave optical links at 4.9 μm wavelength.”,
ACS Photonics, 2018, 5 (9), pp 3689–3694 DOI: 10.1021/acsphotonics.8b00704.
TOP
|
2.3 Al0.25Ga0.75As/GaAs Quantum well infrared photodetectors
on Si substrate using Ge buffer
Al0.25Ga0.75As/GaAs Quantum Well Infrared Photodetectors (QWIPs) were grown by molecular beam epitaxy
on Si substrate. A Ge buffer layer was introduced to reduce threading dislocation density in GaAs caused by the lattice mismatch between
Al0.25Ga0.75As/GaAs and Silicon. Transmission electron microscope images showed good growth quality while preserving good control of the
thickness of quantum wells and barriers. QWIPs grown on both Silicon and GaAs substrates were compared which show similar performances
on the background current, the responsivity and the detectivity. A slightly higher background current observed in the QWIPs on Si
substrate is due to the low-density threading dislocation. The development of high-quality QWIPs on Si substrate is highly promising
for CMOS compatible wide-tunability mid-infrared detection, which would lead to a significant reduction in the cost and an enhanced
production capacity.
|
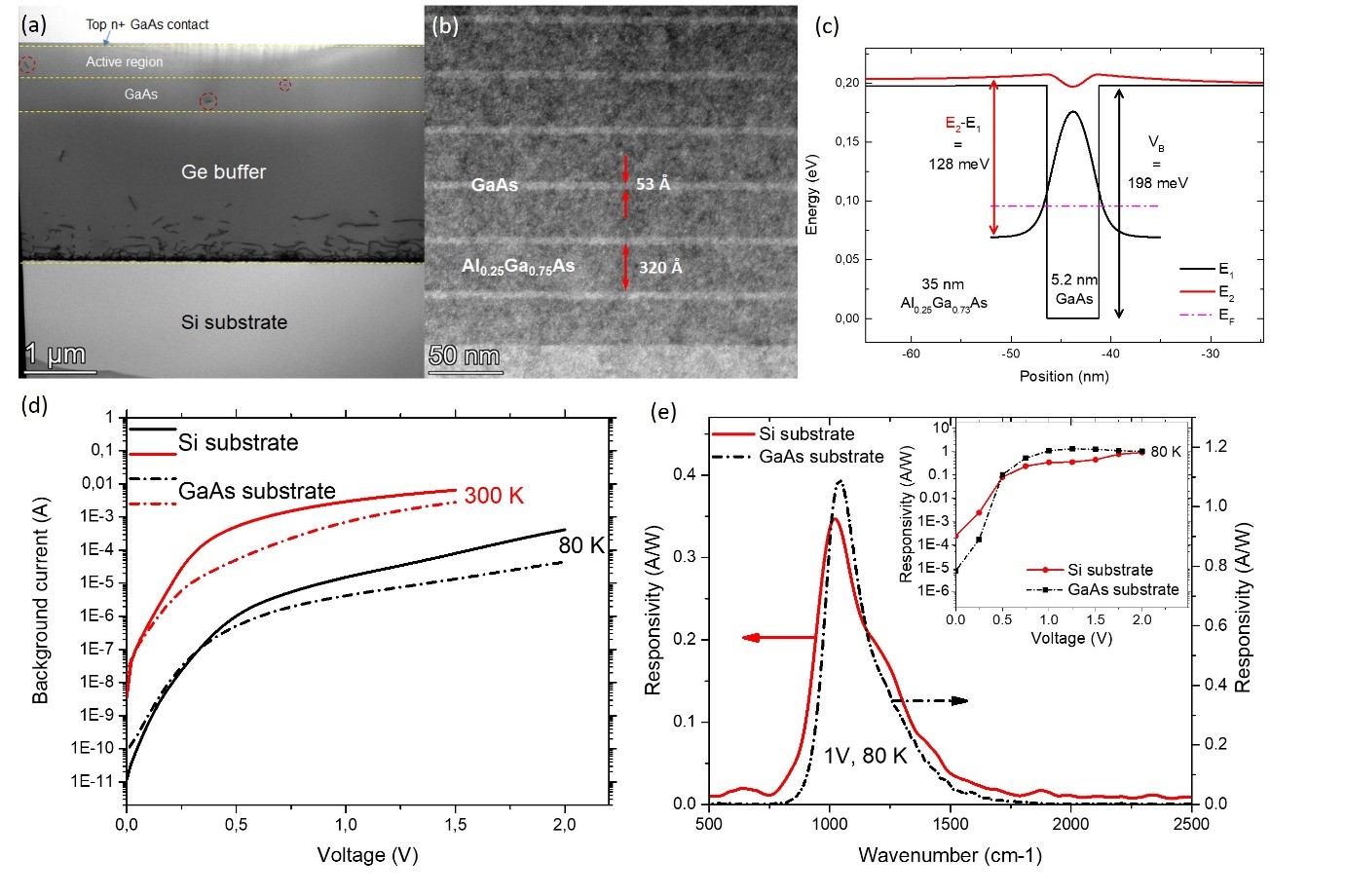
View large figure |
|
|
|
Figure 1.
(a), The Transmission Electron Microscope (TEM) image of Quantum Well Infrared Photodetector (QWIP) grown on Si substrate showing threading dislocations.
Only one dislocation was observed in the active region in this image.
(b), The TEM image of the active region showing the thickness of GaAs and Al0.25Ga0.75As layers.
(c), Band-structure of a single quantum well of the active regions at a bias voltage of 0 V.
The most relevant physical parameters of the structure are indicated in the figure.
(d), Current-voltage (IV) characteristics in background condition at 80 and 300 K.
The solid lines and the short dash dots represent respectively the GaAs substrate and the Si substrate.
(e), responsivity spectra as a function of the wavenumber of the QWIP on Si substrate (solid red line)
and on GaAs substrate (short dash-dot black line) at a bias voltage of 1 Volt and at 80 K. inset: Responsivity as a function of
the voltage of the QWIP on Si substrate (solid red line) and on GaAs substrate (short dash-dot black line) at 80 K.
|
[1] Rodriguez, E., et al. “Al0.25Ga0.75As/GaAs Quantum well infrared photodetectors on Si substrate using Ge buffer.”,
submitted, IEEE Photonics Technology Letters (2019).
|
TOP
3. Monolithic Semiconductor Lasers with Dynamically Tunable Linear-to-Circular Polarization
The ability to control the polarization state of emission from semiconductor lasers is essential
for many applications in spectroscopy, imaging, and communications, inter alia, with monolithic integration approaches being extremely
beneficial. Although manipulating the output polarization of radiation from a laser can be achieved through a number of approaches,
obtaining continuous dynamic control, e.g., from linear to circular, remains extremely challenging. In this paper, we demonstrate that
the polarization of terahertz (THz) frequency radiation can be continuously tuned electronically from linear to circular polarization
by monolithically integrating in-plane metasurfaces with two phase-locked semiconductor-based THz quantum cascade lasers. Moreover, the
metasurfaces—metal antenna arrays in this case—also act as efficient beam collimators, yielding a collimated beam
divergence of~10° ×10°. Our results, however, have broad applicability to a wide range of semiconductor lasers operating
from the visible to THz regions of the electromagnetic spectrum.
|
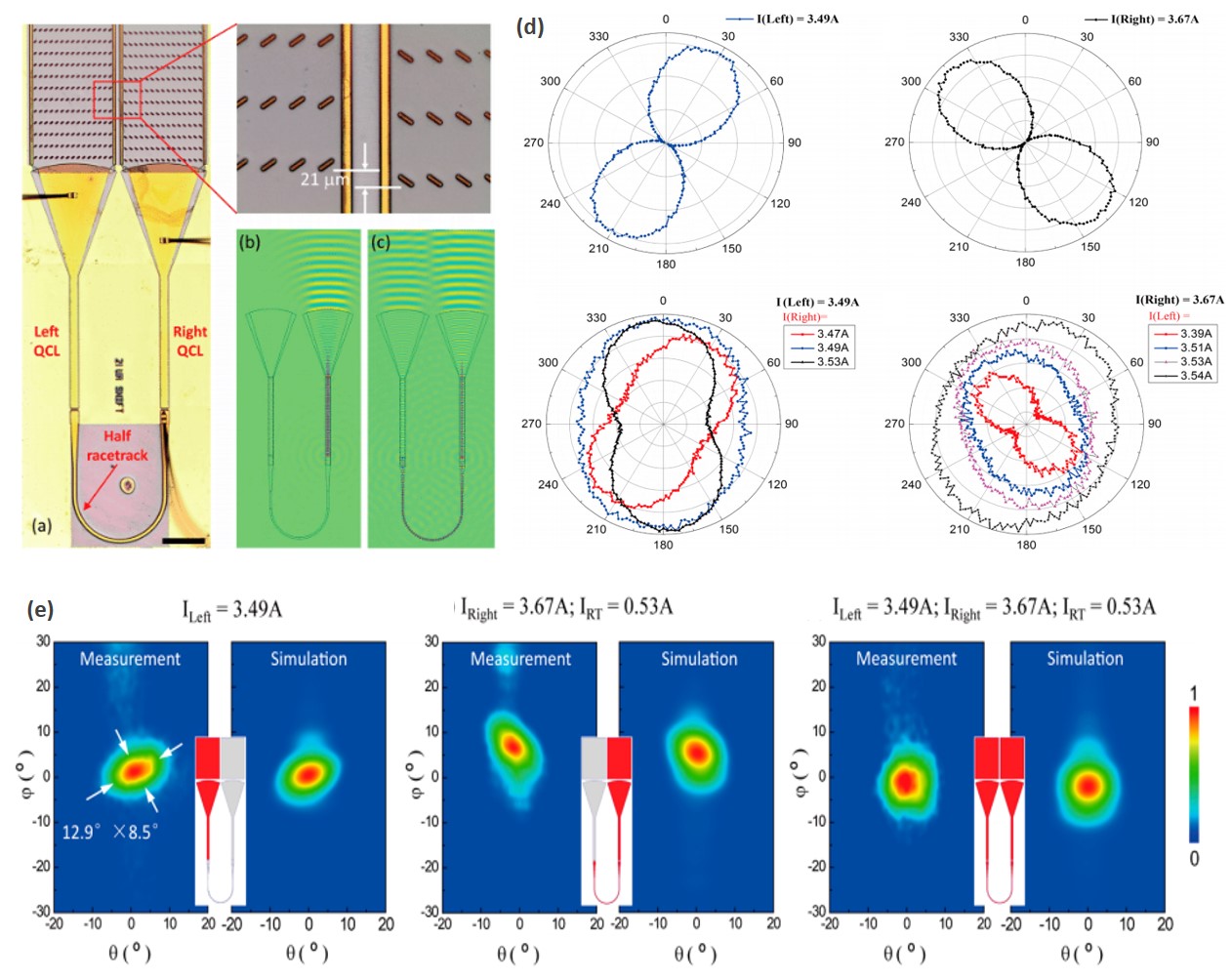
View large figure |
|
|
|
Figure 1. (a) Optical microscope image of a fabricated device. The two sets of antennas are cross oriented and vertically offset by 21μm, corresponding to a~π/2 phase shift. The QCL is designed with a tapered structure to collimate THz light in the lateral direction. The left and right QCLs are phase-locked by a half-racetrack structure to ensure coherent interference of the radiation from the two antenna arrays. The scale bar is 300μm long. (b) Simulated electric field distribution in the device when only the right-hand QCL is electrically pumped. (c) Situation when both QCLs, as well as the half-racetrack structure, are pumped simultaneously, but with different injection currents. The phase-locking scheme allows relatively independent control of the intensity from each QCL. (a) Polarization state of the laser beam at different pumping conditions. This is measured by rotating a wire-grid polarizer in front of the detector. The power for all the measurements is collected at the intensity maximum of the far-field distribution within a half-angle of 2.5°. Evolution of the polarization states when changing the injection current to the QCLs was observed. The device was demonstrated to be capable of dynamically tuning its polarization state in the power collection region from linear to near-circular, with a DOCP value as high as 99%. (e) Measured and simulated far-field patterns of the device with different pumping conditions. Collimated beam divergence of~10° ×10° was always achieved. |
|
|
|
[1] G. Liang, Y. Zeng, X. Hu, H. Yu, H. Liang, Y. Zhang, L. Li, A. G. Davies, E. H. Linfield, and Q. J. Wang*,
"Monolithic Semiconductor Lasers with Dynamically Tunable Linear-to-Circular Polarization",
ACS Photonics 4, 517-524, 2017.
|
TOP
4. Designer Multimode Localized Random Lasing in Amorphous Lattices at
Terahertz Frequencies
Random lasers are a special class of laser in which light is confined through multiple scattering
and interference process in a disordered medium, without a traditional optical cavity. They have been widely studied to investigate
fundamental phenomena such as Anderson localization, and for applications such as speckle-free imaging, benefitting from multiple
lasing modes. However, achieving controlled localized multi-mode random lasing at long wavelengths, such as in the terahertz (THz)
frequency regime, remains a challenge. Here, we study devices consisting of randomly-distributed pillars fabricated from a quantum
cascade gain medium, and show that such structures can achieve transverse-magnetic polarized (TM) multi-mode random lasing, with
strongly localized modes at THz frequencies. The weak short-range order induced by the pillar distribution is sufficient to ensure
high quality-factor modes that have a large overlap with the active material. Furthermore, the emission spectrum can be easily
tuned by tailoring the scatterer size and filling fraction. These “designer” random lasers, realized using standard
photolithography techniques, provide a promising platform for investigating disordered photonics with pre-designed randomness
in the THz frequency range, and may have potential applications such as speckle-free imaging. |
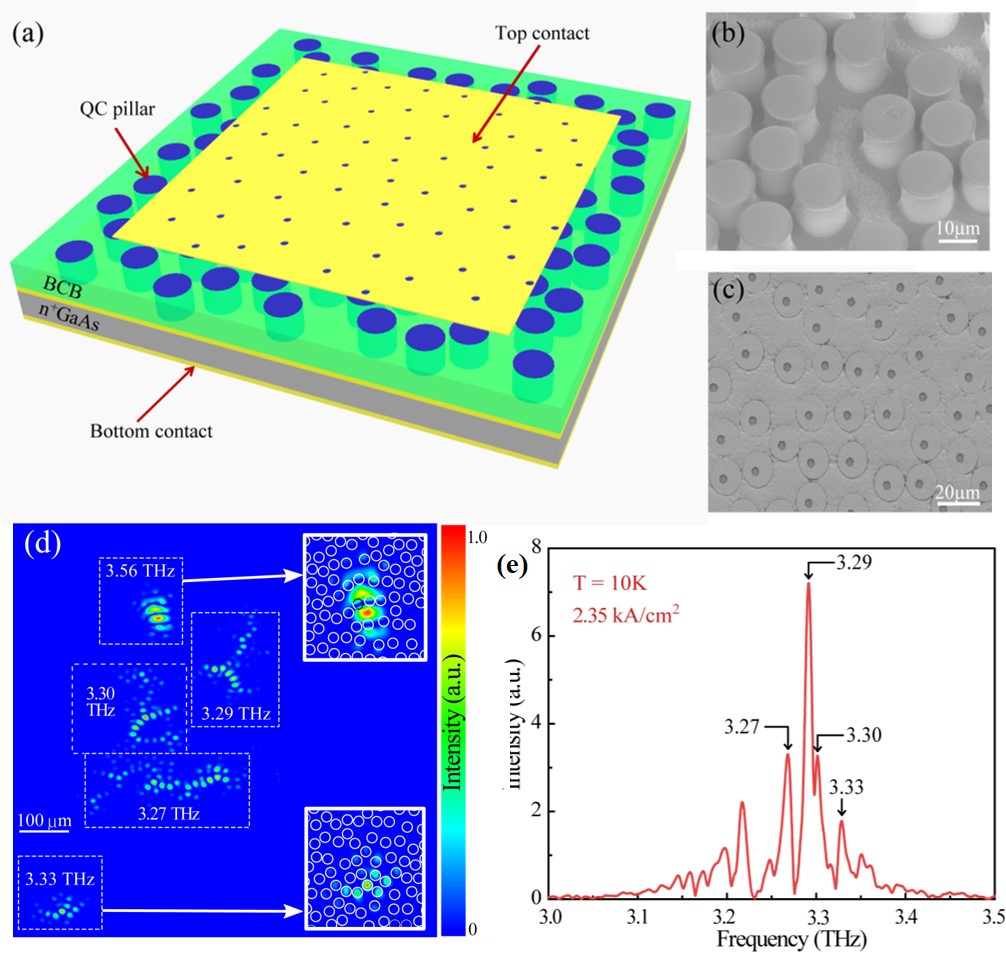
View large figure |
|
|
|
Figure 1. Terahertz random laser with two-dimensional (2D) amorphous pillar lattice. (a) 3D schematic diagram of a surface-emitting terahertz random quantum cascade laser. (b) Scanning electron microscope (SEM) images of the quantum cascade (QC) pillars at an oblique angle after inductively coupled plasma (ICP) dry etching, and (c) the surface of the finished device. The pillar height is 10 µm. Holes of radius 1.5 µm are etched into the top contact plate, at the pillar centers, to allow for surface emission. (d) Field intensity distributions of the modes. The mode intensities have been normalized for clarity. All modes are localized. (e) Emission spectrum of the device at the rollover current density, measured at 10 K. The spectral resolution is 0.2 cm-1. The spectral peaks occur between 3.1–3.4 THz. Some peaks have good frequency match with the calculation. |
|
|
|
Y. Zeng, G. Liang, H. K. Liang, S. Mansha, B. Meng, T. Liu, X. Hu, J. Tao, L. Li, A. G. Davies, E. H. Linfield, Y. Zhang, Y. Chong, and Q. J. Wang*, "Designer Multimode Localized Random Lasing in Amorphous Lattices at Terahertz Frequencies", ACS Photonics 3, 2453−2460, 2016. |
Top
5. Tunable quantum cascade laser
5.1 Surface acoustic wave quantum cascade laser
A novel distributed feedback quantum cascade laser (DFB-QCL) modulated by travelling surface acoustic wave
(SAW) is proposed and theoretically studied. The device is based on a highly piezoelectric Zinc Oxide (ZnO) thin film applied directly on top
of the QCL to enhance the SAW modulation of the device. To increase the coupling efficiency between the optical lasing mode and the SAW-induced
DFB grating, air-waveguide and surface plasmon waveguide structures with two-section active regions are proposed. Simulation results show that a
moderate coupling coefficient of ~2.5 cm-1 can be achieved for the structures, assuming high quality piezoelectric material and device fabrication
can be achieved. The proposed scheme may provide a potentially alternative approach to achieve single-mode, tunable QCLs.
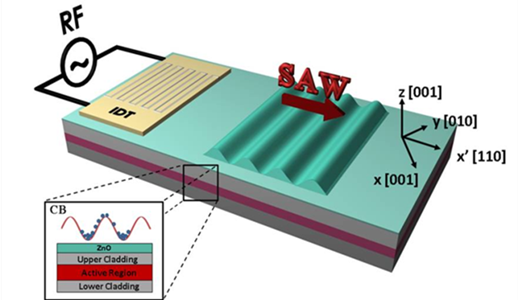
Fig. 1. Schematic diagram of the SAW modulated QCL.
The travelling SAW is generated by applying an RF signal on the integrated interdigital transducer (IDT)
and propagates along the x’[110] crystallographic direction. The inset shows the general waveguide structure and the conduction band (CB) electron
density modulation of QCLs caused by the travelling SAW. A Zinc Oxide (ZnO) thin film is deposited on the top of the waveguide structure to increase
the coupling efficiency.
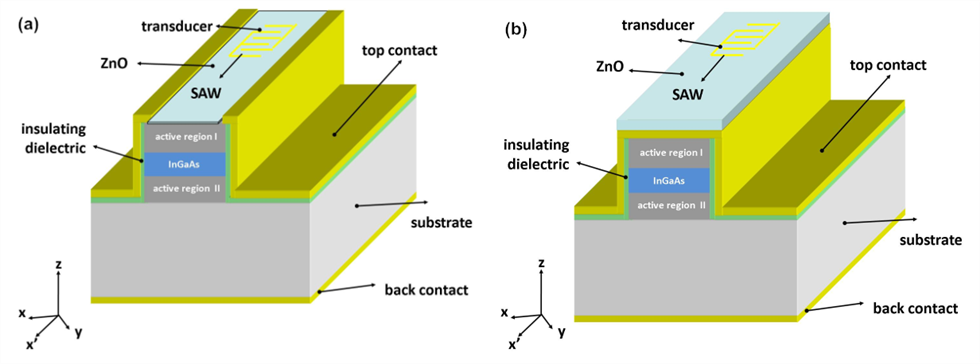
Fig. 2. (a) 3D schematic diagram of the proposed air-waveguide and (b)surface plasmon waveguide structure.
B. Meng, M. Yamanishi, C. Pflugel, Kazuue Fujita, F. Capasso, and Q. J. Wang*, “Investigation of tunable
single-mode quantum cascade lasers via surface-acoustic wave modulation”, IEEE Journal of Quantum Electronics 49, 1053-1061, 2013. |
|
|
|
5.2 Tunable single-mode slot waveguide quantum cascade laser
We report experimental demonstration of tunable, monolithic, single-mode quantum cascade lasers
(QCLs) at ~10 μm with a two-section etched slot structure. A single-mode tuning range of 77 cm-1 (785 nm), corresponding to ~7.8% of the relative tuning range, was realized with a ~20 dB side mode
suppression ratio within the whole tuning range. Compared with integrated distributed feedback
QCLs, our devices have the advantages of easy fabrication and a broader tuning range. Further
theoretical analyses and numerical simulations show that it is possible to achieve a broad continuous
tuning range by optimizing the slot structures. The proposed slot-waveguide design could provide an
alternative but simple approach to the existing tuning schemes for realizing broadly continuous
tunable single-mode QCLs.
|
|
Fig. 1. The microscope image of the slot-QCLs structure. |
Fig. 2. Single-mode evolution of the slot-QCLs. |
B. Meng, J. Tao, X. H. Li, Y. Q. Zeng, S. We, and Q. J. Wang*, “Tunable single-mode slot waveguide quantum cascade lasers”, Applied Physics Letters 104, 201106, 2014.
B. Meng and Q. J. Wang*, “Broadly tunable single-mode mid-infrared quantum cascade lasers”, Journal of Optics 17, 023001, 2015. Invited review. |
|
TOP
|